Introduction
Arboviruses, a broad group of pathogens transmitted by arthropods, pose a significant global health challenge. These viruses, encompassing members from families such as Flaviviridae, Togaviridae, and Bunyavirales, are responsible for a spectrum of human illnesses, ranging from mild febrile conditions to severe and life-threatening diseases like encephalitis and hemorrhagic fevers. Historical accounts suggest the emergence of New World arboviruses, such as yellow fever virus, as early as the 16th century, linked to transatlantic slave trade routes from Africa. Today, viruses like Japanese encephalitis virus (JEV), yellow fever virus (YFV), dengue virus (DENV), West Nile virus (WNV), Zika virus (ZIKV), Crimean-Congo hemorrhagic fever virus (CCHFV), severe fever with thrombocytopenia syndrome virus (SFTSV), and Rift Valley fever virus (RVFV) are recognized as major threats to human health globally.
The imperative for accurate and rapid Arbovirus Diagnosis is driven by the expanding global footprint of these diseases. Factors such as environmental changes, increased international travel, deforestation, urbanization, and lapses in vector control amplify the spread of arboviruses into previously unaffected regions. Effective diagnostic tools are crucial not only for identifying the causative agents of infection but also for implementing timely public health interventions and patient management strategies. While traditional serological methods like Enzyme-Linked Immunosorbent Assays (ELISAs) and Lateral Flow Assays (LFAs) have been foundational in arbovirus diagnosis, the landscape is evolving. Next-Generation Sequencing (NGS) offers powerful surveillance capabilities, but its complexity and cost limit its routine diagnostic utility. Reverse Transcriptase-Polymerase Chain Reaction (RT-PCR) assays, while highly sensitive, typically require centralized laboratory settings due to specialized equipment.
Recent advancements in molecular diagnostics have ushered in an era of isothermal nucleic acid amplification technologies (INAATs). These innovative methods enable nucleic acid amplification at a constant temperature, eliminating the need for thermal cycling. Isothermal amplification techniques hold immense potential for decentralizing arbovirus diagnosis, offering rapid, cost-effective point-of-care (POC) testing, and field-deployable solutions, particularly valuable in resource-constrained environments. This review will delve into the latest progress in isothermal amplification technologies and their application in arbovirus diagnosis, underscoring the future direction of these transformative diagnostic tools.
The urgency for improved diagnostics is underscored by the recurring emergence and re-emergence of infectious diseases. The 20th and 21st centuries have witnessed devastating pandemics and epidemics, including the 1918-1920 influenza pandemic, the ongoing HIV pandemic, and more recent outbreaks of SARS-CoV-1, swine flu, Ebola, Zika, and SARS-CoV-2. These events highlight the critical need for innovative diagnostic tools, especially POC devices, capable of delivering swift and precise detection of emerging pathogens, including arboviruses. The Zika virus serves as a stark reminder; initially considered to cause mild illness, it was later linked to severe conditions like microcephaly and Guillain-Barré syndrome. Experts caution that other flaviviruses, such as Spondweni virus (SPOV) and Usutu virus (USUV), or tick-borne flaviviruses, could potentially emerge as significant human health threats. Similarly, Rift Valley fever virus (RVFV) and alphaviruses like Mayaro virus (MAYV) are considered potential emerging threats due to their geographical spread and adaptive potential. The development and deployment of rapid, sensitive, and affordable POC arbovirus diagnosis tools are therefore paramount, especially in resource-limited settings where diagnostic infrastructure may be lacking.
Arboviral Diseases: Impact on Humans and Animals
Arboviruses represent a diverse group of pathogens capable of infecting a wide range of hosts, including both humans and animals. The majority are RNA viruses, encompassing single-stranded or double-stranded RNA genomes, belonging to families such as Flaviviridae, Togaviridae, Phenuviridae, Peribunyaviridae, Reoviridae, Rhabdoviridae, Orthomyxoviridae, and Gammaentomopoxvirus. African swine fever virus, a DNA virus from the Asfarviridae family, is a notable exception. The arbovirus catalog continues to expand, with ongoing discoveries attributed to advancements in virus isolation and molecular detection techniques.
Transmission of arboviruses is vector-borne, typically through the bite of infected mosquitoes, ticks, or other biting insects. The natural cycle often involves wild animals and birds as reservoirs, with spillover to humans and domestic animals, which often act as dead-end hosts. Arboviral infections in humans and animals can manifest with a broad spectrum of clinical presentations, from asymptomatic infection to severe, life-threatening conditions like hemorrhagic fevers and encephalitis, potentially leading to long-term health complications.
The significant human morbidity and mortality associated with arboviral diseases translate into substantial socio-economic burdens, particularly during outbreaks. Table 1 lists several key arboviruses known to cause human disease. These viruses exhibit a global distribution, with some showing geographical restrictions aligned with the distribution of their specific insect vectors. Mosquitoes are vectors for numerous emerging and re-emerging arboviruses, notably dengue, chikungunya, yellow fever, and Zika viruses. Dengue alone causes a massive global health burden, with estimates of up to 400 million infections and 100 million clinical cases annually. Effective arbovirus diagnosis is therefore crucial in mitigating the impact of these widespread and impactful diseases.
Table 1: Key Human Arboviruses, Vectors, and Symptoms
Virus | Family/Order | Vector | Symptoms | Reference |
---|---|---|---|---|
Zika virus (ZIKV) | Flaviviridae | Aedes mosquitoes | Fever, conjunctivitis, joint pain, headache, maculopapular rash, microcephaly, Guillain–Barré syndrome. | [10] |
Yellow fever virus (YFV) | Flaviviridae | Aedes mosquitoes | Jaundice, liver damage, gastrointestinal bleeding, recurring fever. | [11] |
Dengue Virus (DENV) | Flaviviridae | Aedes mosquitoes | Fever, headache, nausea, muscle and joint pain, skin rash, hypovolemic shock, hemorrhage. | [12] |
West Nile virus (WNV) | Flaviviridae | Culex mosquitoes | Fever, headache, nausea, vomiting, swollen lymph nodes, meningitis, encephalitis, acute flaccid paralysis. | [13] |
Japanese encephalitis virus (JEV) | Flaviviridae | Culex mosquitoes | Mild flu-like symptoms, encephalitis, seizures, paralysis, coma and long-term brain damage. | [14] |
Tick-borne encephalitis virus (TBEV) | Flaviviridae | Ixodes ticks, Dermacentor and Haemaphysalis | Mild meningitis to severe meningoencephalitis with or without paralysis and long-term brain damage damage. | [15] |
Omsk hemorrhagic fever virus (OHFV) | Flaviviridae | Dermacentor ticks | Fever, headache, nausea, muscle pain, cough and hemorrhages. | [16] |
Saint Louis encephalitis virus (SLEV) | Flaviviridae | Culex mosquitoes | Headache, sensory depression, temporal–spatial disorientation, tremors and changes in consciousness. | [17] |
Kyasanur Forest disease virus (KFDV) | Flaviviridae | Haemaphysalis spinigera | Fever with hemorrhagic and/or neurological features in 20% of patients. | [18] |
Chikungunya virus (CHIKV) | Togaviridae | Aedes mosquitoes | Fever frequently associated with joint pain, polyarthralgia and arthritis, rash, myalgia and headache. | [19] |
O’nyong nyong virus (ONNV) | Togaviridae | Anophles mosquitoes | Low-grade fever, symmetrical polyarthralgia, lymphadenopathy, generalized papular or maculopapular exanthema and joint pain. | [20] |
Ross river virus (RRV) | Togaviridae | Culex and Aedes mosquitoes | Arthritis, rash, fever, fatigue and myalgia. | [21] |
Eastern equine encephalitis virus (EEEV) | Togaviridae | Culiseta mosquitoes | Fever, chills, vomiting, myalgia, arthralgia, malaise and encephalitis. | [22] |
Western equine encephalitis virus (WEEV) | Togaviridae | Aedes, Culex and Culiseta mosquitoes | Fever, chills, headache, aseptic meningitis and encephalitis. | [23] |
Venezuelan equine encephalitis virus (VEEV) | Togaviridae | Culex mosquitoes | Fever, chills, malaise, severe headache, myalgia, seizures, drowsiness, confusion and photophobia. | [24] |
Barmah Forest virus (BFV) | Togaviridae | Culex and Aedes mosquitoes | Asymptomatic to relatively mild symptomatic presentations, such as fever and rash; in more severe diseases, polyarthralgia or arthritis. | [25] |
Thogoto virus (THOV) | Orthomyxoviridae | Haemaphysalis and Amblyomma ticks | Benign febrile symptoms to meningoencephalitis. | [26] |
Rift Valley fever virus (RVFV) | Bunyvirales | Culex and Aedes mosquitoes | Fever, headache, backache, vertigo, anorexia, photophobia, hepatitis, jaundice, hemorrhagic disease and ocular complications | [27, 28] |
Ngari virus (NRIV) | Bunyvirales | Aedes, Culex and Anopheles mosquitoes | Fever, joint pain, rash, can induce severe and fatal hemorrhagic fever | [28] |
Severe fever with thrombocytopenia syndrome virus (SFTSV) | Bunyvirales | Haemophysalis Amblyomma, Ixodes and Rhipicephalus ticks | High fever, gastrointestinal symptoms, thrombocytopenia, leukopenia and multiple organ failure | [29] |
Crimean–Congo hemorrhagic fever virus (CCHFV) | Bunyvirales | Hyalomma, Rhipicephalus and Dermacentor ticks | Non-specific febrile illness, sudden onset of fever, myalgia, diarrhea, nausea and vomiting, hemorrhages at various sites around the body. | [30] |
Jamestown Canyon virus (JCV) | Bunyvirales | Aedes, Coquillettidia, Culex mosquitoes | Non-specific febrile illness, meningitis or meningoencephalitis | [31] |
La Crosse encephalitis virus (LACV) | Bunyvirales | Aedes mosquitoes | Fever, headache, myalgia, malaise and occasional prostration, encephalitis and lifelong sequelae. | [32] |
Oropouche Virus (OROV) | Bunyvirales | Culicoides and Culex mosquitoes | Acute febrile illness, myalgia, arthralgia, dizziness, photophobia, rash, nausea, vomiting, diarrhea, conjunctive congestion and meningitis. | [33] |
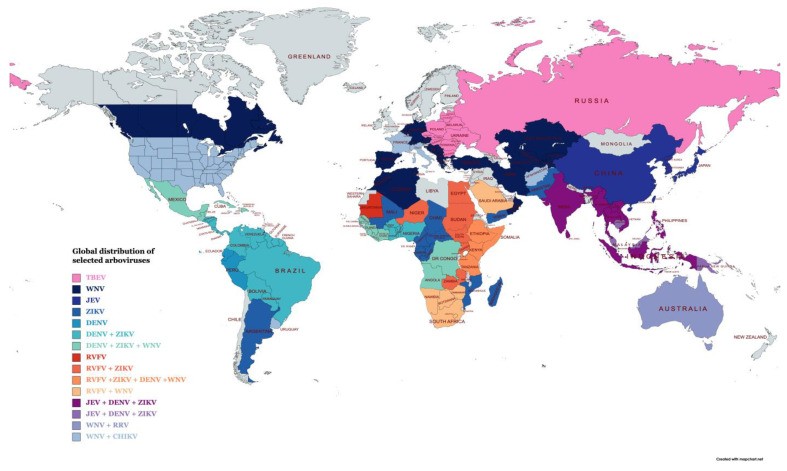
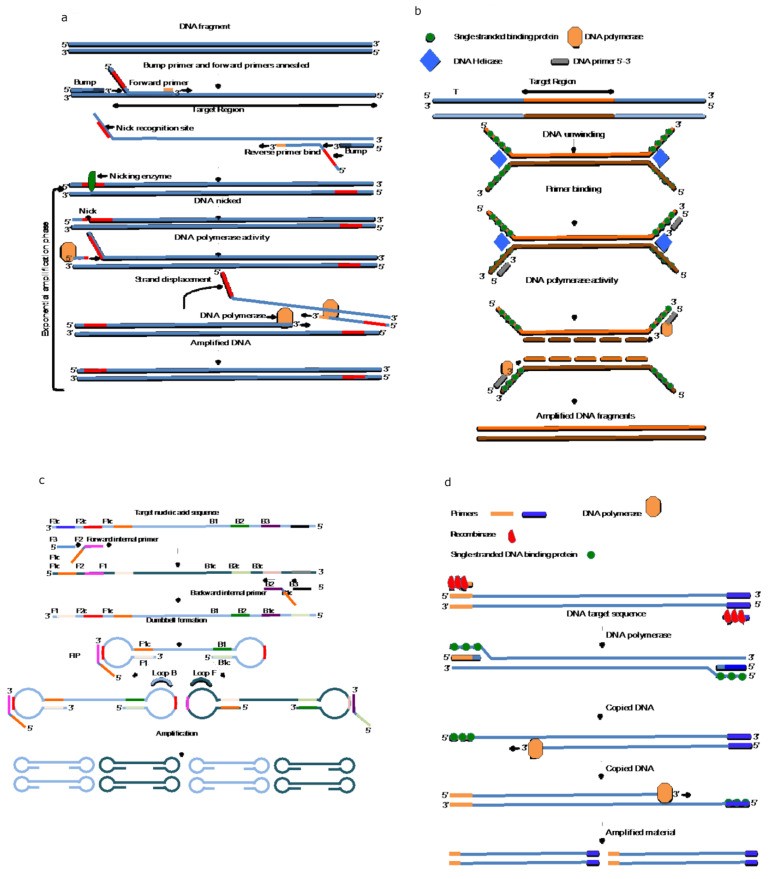
Diagnostic Strategies for Arboviruses
Effective arbovirus diagnosis relies on a range of methodologies, each with its strengths and limitations. These approaches can be broadly categorized into serological assays and molecular techniques, with NGS playing an increasingly important role in surveillance.
Serological Methods in Arbovirus Diagnosis
Historically, serology has been the cornerstone of arbovirus diagnosis. Serological assays encompass direct tests for viral antigen detection and indirect tests that identify host antibodies produced in response to infection. Enzyme-linked immunosorbent assays (ELISAs), particularly IgM capture ELISAs for early infection detection and indirect IgG assays, are widely used. However, cross-reactivity among closely related arboviruses necessitates confirmatory tests like the plaque reduction neutralization test (PRNT), which quantifies neutralizing antibodies in serum or cerebrospinal fluid (CSF). Other serological techniques, including immune magnetic agglutination assays and rapid diagnostic tests (RDTs) such as lateral flow assays, are also utilized in arbovirus diagnosis. Commercial serological kits are available for numerous arboviruses, including dengue, Zika, chikungunya, yellow fever, and Rift Valley fever virus.
Despite their speed and affordability, serological methods have limitations. Cross-reactivity among related viruses, such as Zika, dengue, West Nile, and Japanese encephalitis viruses, can lead to false-positive results. Immunocompromised patients may produce false-negative results due to impaired B-cell responses. Indirect antibody assays also have a “window period” of 3-5 days post-infection before antibodies become detectable, potentially causing false negatives in early-stage infections. Direct antigen detection assays, like NS1 antigen tests for dengue, can address this window period but may suffer from lower sensitivity. Furthermore, serology alone cannot provide serotype information, which is crucial for managing patients with repeat dengue infections. Molecular methods are therefore essential to distinguish specific viruses and serotypes for accurate arbovirus diagnosis and patient care.
Next-Generation Sequencing (NGS) for Arbovirus Detection
Next-Generation Sequencing (NGS) has emerged as a powerful tool in arbovirus diagnosis, particularly in surveillance and outbreak investigations. NGS allows for unbiased sequencing of clinical samples and vector pools, making it invaluable when an unknown pathogen is suspected. Viral metatranscriptomic NGS (mNGS) has been used to survey vectors for arboviruses, revealing the co-circulation of multiple virus types that would be challenging to identify using targeted RT-PCR.
NGS has proven useful in diagnosing arboviral encephalitis/meningitis cases and in patients with symptoms suggestive of arboviral infection but with inconclusive RT-PCR results. It has also been applied to analyze Orthomyxoviridae strains from different geographical regions, aiding in viral classification and phylogenetic studies. In a febrile illness survey in Colombia, mNGS identified Oropouche virus (OROV) circulation in RT-PCR-negative samples, highlighting OROV as an emerging pathogen in the region. mNGS has also been used for Orbivirus detection in Japanese mosquitoes and mosquito species identification in Mexico.
While mNGS excels in broad viral surveillance, methods for enriching specific viral sequences in samples, such as vector pools, have been developed. Viral-specific enrichment has been successfully used to characterize Crimean-Congo hemorrhagic fever virus (CCHFV) and Jingmen virus (JMTV) in pooled tick samples and to detect unknown Ross River virus circulation in Australian arbovirus surveillance programs.
Despite significant cost reductions and increased accessibility, NGS remains relatively expensive and requires specialized equipment, bioinformatics infrastructure, and highly trained personnel. Its complexity and turnaround time currently limit its application in point-of-care arbovirus diagnosis and resource-limited settings for routine screening.
Reverse Transcriptase PCR (RT-PCR) in Arbovirus Diagnosis
Reverse Transcriptase PCR (RT-PCR) has become the gold standard molecular method for arbovirus diagnosis, offering enhanced sensitivity and specificity compared to serological and NGS methods (without viral enrichment). RT-PCR is widely used for diagnosing various viral infections, including respiratory, gastrointestinal, and tropical diseases. It is applicable to a broad spectrum of human and animal arboviral infections.
However, conventional RT-PCR is generally not suitable for point-of-care (POC) applications due to the requirement for thermal cycling equipment, specialized infrastructure, and trained staff. Novel RT-PCR technologies, such as 3base™ technology, are being developed to simplify the process and potentially enhance pan-family detection. 3base™ technology, based on bisulphite conversion of cytosine to thymine, reduces the complexity of nucleic acid sequences, making viral family genomes more similar at the nucleic acid level. This facilitates the design of pan-family PCR primers and probes, enabling broader detection of arboviral families like flaviviruses and alphaviruses. While improving surveillance capabilities, traditional RT-PCR remains largely confined to centralized laboratories for arbovirus diagnosis.
Sample-to-Answer RT-PCR Systems
Developments in sample-to-answer RT-PCR systems aim to streamline the diagnostic process, reducing hands-on time and complexity. These systems integrate sample preparation, amplification, and detection into a single platform. While offering improvements in workflow and accessibility, many still rely on thermal cycling and may not fully address the need for low-cost, field-deployable arbovirus diagnosis solutions.
Isothermal Amplification Technologies: Revolutionizing Arbovirus Diagnosis
Isothermal Nucleic Acid Amplification Technologies (INAATs) are transforming the landscape of arbovirus diagnosis. These methods amplify nucleic acids at a constant temperature, eliminating the need for thermal cyclers and opening up new possibilities for point-of-care and decentralized testing. Several INAAT methods have emerged, each with unique mechanisms and advantages.
Strand Displacement Amplification (SDA) and Nicking Endonuclease Amplification Reaction (NEAR)
Strand Displacement Amplification (SDA), developed in 1992, relies on a DNA polymerase with 5′-3′ exonuclease activity and a specific restriction enzyme to nick and displace DNA strands, leading to exponential amplification at a constant temperature. A simplified and rapid version, Nicking Endonuclease Amplification Reaction (NEAR), developed by Abbott Laboratories, amplifies RNA in as little as 10-13 minutes. NEAR has been implemented in sample-to-answer devices for rapid SARS-CoV-2 detection, demonstrating its potential for POC and field-based arbovirus diagnosis. The technology achieves high amplification efficiency (109-fold) in under 15 minutes, making it highly suitable for rapid, decentralized testing.
Nucleic Acid Sequence-Based Amplification (NASBA)
Nucleic Acid Sequence-Based Amplification (NASBA), an early isothermal amplification technology developed in 1991, employs a three-enzyme system (AMV reverse transcriptase, RNase H, and T7 RNA polymerase) to amplify RNA targets. NASBA operates at 41°C and achieves 106–109-fold amplification in 60–90 minutes. The reaction produces RNA amplicons, which can be detected using gel electrophoresis, fluorescent probes in real-time NASBA, or colorimetric techniques like NASBA-ELISA. NASBA is particularly useful for RNA virus detection in arbovirus diagnosis, although it is specific to RNA targets.
Helicase-Dependent Amplification (HDA)
Helicase-Dependent Amplification (HDA) utilizes the intrinsic unwinding activity of helicase enzymes to separate double-stranded DNA for primer annealing and subsequent DNA polymerase-mediated amplification. HDA operates isothermally between 37°C and 65°C, achieving 106-fold amplification in 30–90 minutes. HDA is adaptable to colorimetric, fluorescent, and lateral flow readouts, making it suitable for POC and field-based arbovirus diagnosis. HDA has been successfully used to detect a range of pathogens, including Norovirus, SARS-CoV-2, M. tuberculosis, T. vaginalis, and yellow fever virus in resource-limited settings.
Loop-Mediated Isothermal Amplification (LAMP)
Loop-Mediated Isothermal Amplification (LAMP), introduced in 2000, is a widely used INAAT method for both DNA and RNA amplification. LAMP uses Bacillus stearothermophilus DNA Polymerase I (Bst DNA polymerase), which exhibits both DNA polymerase and strand displacement activities. LAMP employs 4-6 target-specific primers for rapid and efficient amplification at 65°C. Next-generation polymerase enzymes are under development for ambient temperature LAMP, further simplifying POC arbovirus diagnosis. LAMP is renowned for its speed, achieving 109-fold amplification from low copy targets in as little as 60 minutes, and often even faster. Positive LAMP reactions can be visually detected via turbidity or color change, simplifying readout. LAMP enzymes are also more tolerant to inhibitors in biological samples compared to Taq polymerase. These features make LAMP an ideal platform for POC and field-deployable arbovirus diagnosis, particularly in resource-limited settings.
Various readout methods enhance LAMP’s versatility. Turbidity detection, colorimetric detection using dyes like hydroxy napthol blue or commercial colorimetric master mixes, and fluorescent probes are all compatible with LAMP. Furthermore, coupling LAMP with CRISPR-Cas12 enzyme systems and lateral flow detection offers highly sensitive and rapid arbovirus diagnosis solutions suitable for field deployment.
Recombinase Polymerase Amplification (RPA) and Recombinase Aided Amplification (RAA)
Recombinase Polymerase Amplification (RPA), described in 2006, employs recombinase-primer complexes to initiate strand exchange at target sites, destabilizing DNA duplexes and enabling strand displacement and polymerase-mediated DNA synthesis. RPA, also known as Recombinase Aided Amplification (RAA), uses strand-displacing DNA polymerases like Bst DNA polymerase, similar to LAMP. RPA operates at 37°C to 42°C and achieves 107–108-fold amplification in approximately 60 minutes, with recent advancements reducing amplification times to as little as 3-15 minutes. RPA is compatible with lateral flow and fluorescent detection and, like LAMP, exhibits tolerance to inhibitors in primary samples, potentially reducing the need for complex sample processing in arbovirus diagnosis. RPA has been used for detecting Rift Valley fever virus, F. tularensis, HIV, biothreat agents, and yellow fever virus. Coupling RPA with CRISPR-Cas12 systems and lateral flow or fluorescent readouts further enhances its utility for rapid arbovirus diagnosis, including multiplex detection of West Nile virus, Zika, and dengue serotypes.
Isothermal Amplification: A Key Tool in Arbovirus Diagnostics
As discussed, RT-PCR is the established gold standard for molecular infectious disease diagnostics, including arbovirus diagnosis. However, isothermal amplification technologies like LAMP, RPA, SDA, and NASBA offer compelling advantages, particularly for point-of-care and resource-limited settings.
Table 3 summarizes the key features of isothermal amplification techniques compared to conventional RT-PCR. RT-PCR offers high specificity and sensitivity but requires thermal cycling equipment, trained staff, and dedicated infrastructure. INAAT methods, in contrast, eliminate the need for thermal cyclers, enabling simpler, more decentralized arbovirus diagnosis.
Table 3: Comparison of Isothermal Amplification Techniques and RT-PCR
Amplification Method | Template | Temperature | Primers | Time to Result | Enzymes (RNA) | Advantages | Disadvantages |
---|---|---|---|---|---|---|---|
Real-time PCR | DNA/RNA | Thermal cycling | 2 | 15–60 min | 2 | Established method, high sensitivity and specificity; multiplexing capabilities. | Thermal cycler required; highly trained staff needed. |
Nucleic-Acid-Sequence-Based Amplification (NASBA) | RNA | 41 °C | 2 | 90–120 min | 3 | Isothermal, rapid. | RNA-based amplification only. |
Strand Displacement Amplification (SDA) | DNA/RNA | 37–65 °C | 4 | 10–60 min | 3 | Isothermal, rapid results with NEAR method. | Sensitivity can be lower than other methods; thermal denaturation required for DNA. |
Helicase Dependant Amplification (HDA) | DNA/RNA | 37–65 °C | 2 | 30–60 min | 3 | Isothermal, rapid, POC compatible. | Not as sensitive as other techniques. |
Loop mediated AMPlification (LAMP) | DNA/RNA | 65 °C | 4–6 | 5–30 min | 2 | Isothermal, rapid, POC adaptable; multiplex capability; visual detection possible. | Complex primer design. |
Recombinase Polymerase Amplification (RPA) | DNA/RNA | 37–42 °C | 2 | 5–30 min | 3 | Isothermal, rapid, POC compatible. | Reagents currently for Research Use Only (RUO). |
Each INAAT method employs a distinct amplification mechanism to achieve exponential nucleic acid amplification. NASBA is uniquely RNA-specific, while other methods can amplify both RNA and DNA. NASBA, HDA, and RPA require only two primers, similar to RT-PCR, whereas SDA uses four, and LAMP is the most complex, requiring 4-6 primers. LAMP primer design can be simplified using online tools.
INAAT methods, particularly LAMP, RPA/RAA, and NEAR, offer rapid amplification, often within 5-30 minutes, crucial for POC and field-deployable arbovirus diagnosis. These methods are also readily adaptable to low-cost readouts like lateral flow devices (LFDs). A potential drawback of some INAATs, especially LAMP, is the risk of non-specific amplification due to the large amplicon production. SDA requires initial thermal DNA denaturation and may exhibit lower sensitivity compared to other INAATs.
Point-of-Care Diagnostics: Bringing Arbovirus Diagnosis to the Front Lines
The World Health Organization (WHO) has established ASSURED (Affordable, Sensitive, Specific, User-friendly, Rapid and robust, Equipment-free) and REASSURED (additionally: Real-time connectivity, Ease of specimen collection, Enabling access and equity, Sustainable, User-friendly, Rapid and robust, Equipment-free, and Deliverable to end-users) criteria for ideal POC diagnostics, particularly for resource-limited regions. INAAT technologies are well-suited to meet these criteria for arbovirus diagnosis. Several promising POC platforms are being developed in conjunction with INAAT, including lab-on-a-chip, lab-on-a-disc, microfluidic paper-based devices, lateral flow devices, and CRISPR-Cas-based diagnostics.
Lab-on-a-Chip (LOC) Devices for Arbovirus Diagnosis
Lab-on-a-chip (LOC) devices integrate all diagnostic steps, from sample preparation to detection and readout, onto a single microfluidic platform. LOCs are often fully automated, with reagents pre-loaded or in easily inserted cartridges. LOC devices have been developed for arbovirus diagnosis, including Zika virus detection. Examples include battery-operated field-deployable Zika detectors, microfluidic LAMP-based Zika detection platforms, disposable saliva-based Zika detection cassettes, and smartphone-integrated microfluidic LAMP cards for multiplex detection of Zika, dengue, and chikungunya from whole blood. LOC technology offers significant promise for decentralized arbovirus diagnosis in resource-limited settings and POC applications.
Lab-on-a-Disc (LOAD) Platforms in Arbovirus Testing
Lab-on-a-Disc (LOAD) systems, similar to LOCs, integrate sample processing, amplification, and detection, but utilize centrifugal forces, often in combination with microfluidics. A commercial LOAD system, LIASON®MDX, is available for detecting various pathogens, including dengue. INAAT-based LOAD devices are being developed for arbovirus diagnosis, such as a portable LOAD system for highly pathogenic avian influenza virus detection and a low-cost centrifugal microfluidic LOAD for Rift Valley fever virus and yellow fever virus detection. The FeverDisc LOAD system represents a fully integrated LAMP-based platform capable of detecting multiple pathogens, including Plasmodium species and arboviruses (chikungunya, dengue, Zika), with minimal hands-on time and lyophilized reagents for cold-chain independence. LOAD systems offer a comprehensive solution for rapid and decentralized arbovirus diagnosis.
Microfluidic Paper-Based Analytical Devices (µPADs) for Field-Based Diagnostics
Microfluidic Paper-based Analytical Devices (µPADs) are simple, inexpensive, and portable platforms for POC diagnostics. µPADs utilize patterned paper with microchannels to guide sample and reagent flow, enabling reagent storage, mixing, and detection without external power sources. µPADs have been adapted for arbovirus diagnosis, including Zika NS1 protein detection and laser-cut glass fiber µPADs for rapid chikungunya IgM detection. A µPAD-LAMP device concept for SARS-CoV-2 detection, employing wax barriers to compartmentalize reaction zones, demonstrates the potential of µPADs for cost-effective and field-deployable arbovirus diagnosis.
Lateral Flow Devices (LFDs) for Rapid Arbovirus Diagnosis at the Point-of-Care
Lateral Flow Devices (LFDs) are widely used POC platforms due to their low cost, ease of use, and rapid results. While traditional LFDs may lack sensitivity and specificity compared to molecular methods, combining LFDs with isothermal amplification enhances their performance for arbovirus diagnosis. LFDs coupled with HDA, LAMP, and RPA have been developed for detecting various pathogens, including T. vaginalis, biothreat agents, Mycobacterium tuberculosis, Influenza, Japanese encephalitis virus, Zika, SARS-CoV-2, Monkeypox, African swine fever virus, and Heartland virus. LFD-INAAT combinations provide a rapid, user-friendly, and sensitive approach for POC arbovirus diagnosis.
CRISPR-Cas12/13 Technology for Enhanced Arbovirus Detection
CRISPR-Cas technology, leveraging the sequence-specific nucleic acid cleavage activity of CRISPR proteins, offers highly sensitive and specific pathogen detection. CRISPR-Cas12/13 systems, distinct from CRISPR-Cas9, utilize guide RNAs (crRNA) to target specific sequences and activate collateral cleavage of reporter molecules, generating a detectable signal. Coupling CRISPR-Cas systems with INAAT methods like LAMP, RPA, HDA, and SDA creates highly sensitive, rapid, and field-deployable assays for arbovirus diagnosis. CRISPR-Cas-INAAT assays have been developed for detecting dengue, West Nile virus, Zika, Japanese encephalitis virus, Crimean-Congo hemorrhagic fever virus, and severe fever with thrombocytopenia syndrome virus. These CRISPR-enhanced INAAT assays represent a cutting-edge approach for advanced POC arbovirus diagnosis.
Table 4: Comparison of Key Technologies for Arbovirus Diagnosis
| Method | Cost | Ease of Use | Sensitivity | Specificity | POC Applicable | Advantages