Bovine Respiratory Disease (BRD) remains a significant health challenge in the cattle industry, leading to substantial economic losses due to treatment costs, reduced productivity, and mortality. Accurate and timely Bovine Respiratory Disease Diagnosis is crucial for effective management and control strategies, including targeted antimicrobial therapy and preventative measures. This article provides an in-depth overview of current diagnostic methods, interpretation challenges, and best practices for diagnosing BRD in cattle, aiming to equip veterinarians with the knowledge to optimize their diagnostic approaches.
Understanding Sampling Techniques for BRD Diagnosis
Selecting the appropriate sampling method is a critical first step in bovine respiratory disease diagnosis. The choice of technique influences the type and quality of sample obtained, which in turn affects the accuracy and interpretation of diagnostic test results. Several sampling techniques are available, each with its own advantages and disadvantages, summarized in Table 1.
Table 1: Overview of Respiratory Tract Sampling Techniques in Cattle
Technique | Sampling Site | Representative of Lower Airways | Sampled Surface Area | Procedure Cost | Procedure Time (min) | Contamination Risk | Technique Difficulty | Complications |
---|---|---|---|---|---|---|---|---|
Nasopharyngeal Swab (DNS) | Nasopharyngeal mucosa | ± | 2 cm² | Low | 10 | High | Low | Nasal hemorrhage, swab fracture |
Transtracheal Wash (TTW) | Tracheal bifurcation | Yes | 5–10 cm² | Medium | 1-10 | Absent | Medium | Subcutaneous emphysema, wound infection, local hemorrhage, catheter tearing, respiratory distress |
Non-endoscopic BAL (nBAL) | Random lung lobe | Yes, controversial | >10 cm² | Medium | 10 | Moderate | Medium | Nasal hemorrhage, intrapulmonary hemorrhage, airway perforation (rigid catheter), respiratory distress |
Endoscopic BAL (eBAL) | Targeted lung lobe(s) | Yes | >10 cm² | High | 10+ | Low | High | Nasal hemorrhage, respiratory distress |
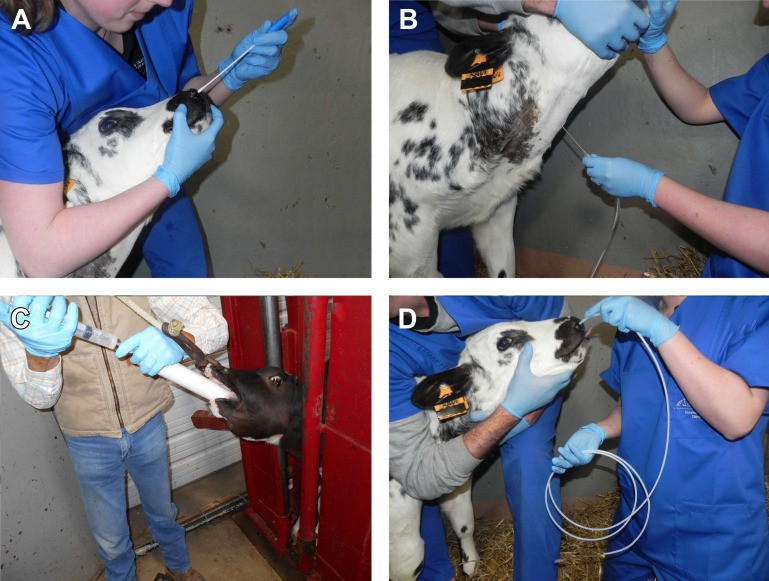
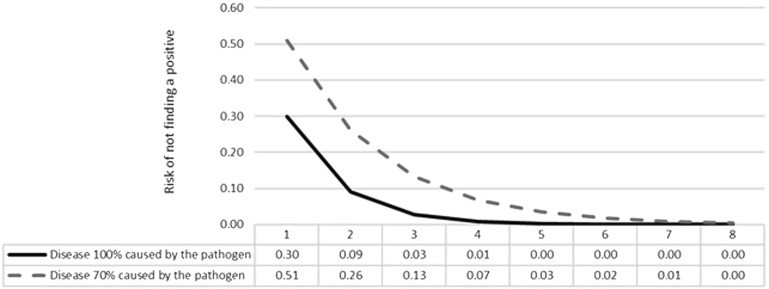
Nasopharyngeal Swabs (DNS) are a minimally invasive and relatively easy-to-perform technique, making them practical for field use. They sample the nasopharyngeal mucosa, which is the initial site of infection for many respiratory pathogens. However, DNS samples are prone to contamination from the upper respiratory tract, and the high prevalence of polymicrobial samples can complicate interpretation, especially when opportunistic pathogens are identified. Despite these limitations, DNS can be useful for detecting primary viral pathogens and certain bacterial pathogens associated with BRD.
Transtracheal Wash (TTW) is considered a more invasive but valuable technique for obtaining samples directly from the lower respiratory tract, bypassing the nasal passages and reducing contamination. TTW involves percutaneous puncture of the trachea and instillation of sterile saline, followed by aspiration of the fluid. This method is particularly useful for bacterial culture and cytologic examination of the lower airways. However, TTW requires more technical skill and carries a risk of complications, such as subcutaneous emphysema and local hemorrhage.
Non-endoscopic Bronchoalveolar Lavage (nBAL) is a less invasive alternative to endoscopic BAL, performed by blindly introducing a catheter through the nasal passages into the lower airways. nBAL allows sampling of a larger area of the lung compared to TTW and provides a representative sample of the distal airways and alveoli. While nBAL is associated with a moderate risk of nasal contamination, studies have shown good agreement with TTW and eBAL for bacterial culture results. nBAL is a practical field technique for collecting samples for both bacterial and viral diagnostics.
Endoscopic Bronchoalveolar Lavage (eBAL) is the gold standard for lower respiratory tract sampling, allowing direct visualization of the airways and targeted sampling of specific lung lobes, particularly those identified as affected by imaging techniques like radiography or ultrasonography. eBAL minimizes contamination risk and provides high-quality samples for cytology, bacteriology, and virology. However, eBAL requires specialized equipment, expertise, and is more time-consuming and costly, limiting its routine use in field settings.
Figure 1: Overview of accessible sampling methods of the airways in cattle. (A) DNS, (B) TTW, (C) nBAL through the mouth under visual control; (D) nBAL performed blindly through the nose.
Diagnostic Tests for Identifying BRD Pathogens
Once a respiratory sample is collected, various diagnostic tests can be employed to identify the causative agents of BRD. These tests can be broadly categorized into culture-based methods, molecular techniques, and serology, each offering unique advantages and limitations in bovine respiratory disease diagnosis (Table 2).
Table 2: Diagnostic Tests for Bacterial Pathogen Detection in BRD
Test | Detects | Turnaround Time (TAT) | Advantages | Disadvantages |
---|---|---|---|---|
Microbial Culture | Live bacteria | 24 h – >5 d | Cheap, live pathogen detection, quantification possible, antimicrobial susceptibility testing (antibiogram) | Live organisms required, time-consuming, lower sensitivity, fastidious bacteria overgrowth, specific media needed |
Polymerase Chain Reaction (PCR) | DNA | 24 h | High sensitivity, no live organisms needed, rapid, detection of multiple pathogens, pooling possible, quantification possible (qPCR) | Possible detection of insignificant quantities or dead bacteria, potential false-positive from vaccine antigen, more expensive |
Serology (ELISA) | Antibodies | 24 h – 3 wk | Useful for herd-level diagnostics, vaccination program monitoring | Indirect evidence of infection, long TAT (3 weeks for paired sera), variable sensitivity and specificity, cannot differentiate vaccine-induced antibodies from infection |
Culture-enriched Direct MALDI-TOF MS | Live bacteria | 6 h – 3 d | Rapid, relatively cheap, antibiogram possible with MBT-ASTRA | MALDI-TOF MS required, lower accuracy in polymicrobial samples |
Nanopore Sequencing (NGS) | DNA (whole genome) | 1-2 d | Comprehensive pathogen detection and quantification, strain typing possible, detection of novel pathogens | No classic antibiogram, more expensive, complex data analysis |
Microbial Culture remains a cornerstone of bovine respiratory disease diagnosis, particularly for bacterial pathogens. Culture allows for the isolation and identification of live bacteria, providing definitive evidence of infection. Moreover, bacterial isolates obtained from culture can be subjected to antimicrobial susceptibility testing, guiding targeted therapy decisions. However, culture is time-consuming, requires specific growth media for certain pathogens (e.g., Mycoplasma), and may have lower sensitivity compared to molecular methods, especially for fastidious or slow-growing organisms.
Polymerase Chain Reaction (PCR) has become increasingly popular in veterinary diagnostics due to its high sensitivity, rapid turnaround time, and ability to detect multiple pathogens simultaneously. Multiplex PCR assays can detect a panel of common viral and bacterial pathogens associated with BRD in a single reaction. Quantitative PCR (qPCR) provides information on pathogen load, which can aid in differentiating colonization from active infection. However, PCR detects DNA, not necessarily live organisms, and may yield false-positive results due to detection of residual DNA from dead bacteria or vaccine antigens. Interpretation of PCR results requires careful consideration of the clinical context.
Serology, specifically antibody-based enzyme-linked immunosorbent assays (ELISAs), plays a limited role in acute bovine respiratory disease diagnosis for individual animals due to the delayed antibody response. However, serology is valuable for herd-level diagnostics, assessing vaccination status, and monitoring disease dynamics over time. Paired serum samples collected 2-3 weeks apart are typically required to demonstrate seroconversion and confirm recent infection. Serology cannot differentiate between vaccine-induced and naturally acquired antibodies.
Matrix-Assisted Laser Desorption/Ionization Time-of-Flight Mass Spectrometry (MALDI-TOF MS) is a rapid and cost-effective technology for bacterial identification based on unique protein profiles. Culture-enriched direct MALDI-TOF MS combines the specificity of culture with the speed of MALDI-TOF MS, significantly reducing turnaround time for bacterial identification compared to traditional culture and biochemical testing. MALDI-TOF MS can also be coupled with rapid antimicrobial susceptibility testing methods like MBT-ASTRA, further accelerating diagnostic workflows.
Next-Generation Sequencing (NGS), including nanopore sequencing technologies like MinION, represents a cutting-edge approach to bovine respiratory disease diagnosis. NGS allows for comprehensive metagenomic analysis of respiratory samples, enabling the detection of all viral and bacterial pathogens present, including novel or uncharacterized agents. NGS provides semi-quantitative data on pathogen abundance and facilitates strain typing, virulence gene detection, and antimicrobial resistance prediction. While currently more expensive and complex than other methods, NGS holds immense promise for advancing our understanding and diagnosis of BRD in the future.
Interpreting Diagnostic Test Results for BRD
Interpreting diagnostic test results in bovine respiratory disease diagnosis is often challenging, particularly when dealing with opportunistic pathogens and polymicrobial infections. A comprehensive interpretation requires integrating information on the pathogen identified, sampling site, diagnostic test used, clinical presentation of the animal, and herd history.
Differentiating Primary and Secondary Pathogens
A crucial step in result interpretation is to distinguish between primary and secondary (opportunistic) pathogens. Primary pathogens, such as Bovine Respiratory Syncytial Virus (BRSV), Bovine Herpesvirus-1 (BHV-1), Bovine Viral Diarrhea Virus (BVDV), and Mycoplasma bovis, are capable of initiating respiratory disease on their own. Their detection in respiratory samples, especially from the lower airways, is generally considered clinically significant.
Secondary pathogens, including Mannheimia haemolytica, Pasteurella multocida, and Histophilus somni, are often part of the normal respiratory flora and require predisposing factors, such as viral infection or stress, to cause disease. The detection of secondary pathogens alone, particularly in upper respiratory tract samples like DNS, should be interpreted cautiously, as they may represent colonization rather than active infection.
Understanding Contamination, Colonization, and Infection
When interpreting bacterial culture results, it is essential to differentiate between contamination, colonization, and infection. Contamination refers to the presence of bacteria in a sample due to improper collection or handling, not reflecting the true microbial flora of the respiratory tract. Colonization is the presence of bacteria in the respiratory tract without causing disease, while infection involves bacterial invasion and inflammation of the respiratory tissues.
Quantitative culture, which assesses the abundance of bacterial growth, can help distinguish between colonization and infection. High bacterial loads, especially of known opportunistic pathogens in lower respiratory tract samples, are more suggestive of infection. Cytologic examination of respiratory samples, revealing neutrophilic inflammation and evidence of bacterial phagocytosis, can further support a diagnosis of bacterial infection.
Sampling Strategy and Group Diagnosis
The diagnostic approach for BRD often involves sampling a subset of animals within an affected group to infer the likely causes and guide herd-level management decisions. Selecting animals in the early stages of disease, prior to antimicrobial treatment, and avoiding severely distressed animals optimizes the diagnostic yield and minimizes potential complications.
Pooling samples from multiple animals (e.g., up to 5) for PCR testing can enhance the sensitivity of group diagnosis, increasing the likelihood of detecting pathogens present within the herd. However, pooling may dilute samples and reduce the quantitative information available from qPCR.
Figure 2: Risk (probability ranging between 0 and 1) of not finding a positive animal for a given pathogen according to sample size (x axis) in a scenario where 100% (solid line) and 70% (dashed line) of affected animals are positive for a given pathogen. The graph represents a test with 70% sensitivity and 100% specificity.
Conclusion: Navigating the complexities of BRD Diagnosis
Bovine respiratory disease diagnosis is a complex and evolving field. While significant advancements have been made in diagnostic technologies, interpretation of results remains challenging due to the multifactorial nature of BRD, the role of opportunistic pathogens, and limitations of current diagnostic tests. Veterinarians must carefully consider the clinical context, sampling technique, diagnostic test employed, and potential for contamination or colonization when interpreting BRD diagnostic results. Integrating clinical findings, herd history, and laboratory data is essential for accurate bovine respiratory disease diagnosis and informed decision-making in BRD management and control. Continued research into the respiratory microbiome, host-pathogen interactions, and novel diagnostic approaches is crucial for further improving our ability to diagnose and effectively manage BRD in cattle.