1. Introduction to Cholangiocarcinoma Diagnosis
Cholangiocarcinoma (CCA), a malignancy originating from the bile duct epithelium, is recognized as the second most common primary liver cancer after hepatocellular carcinoma (HCC). Characterized by a rising global incidence, early and accurate diagnosis of CCA remains challenging yet crucial due to its aggressive nature. Anatomically, CCAs are classified based on their location into intrahepatic, perihilar, and extrahepatic types [1]. Perihilar CCA, also known as Klatskin tumors, is the most frequent, accounting for 50–60% of cases, followed by extrahepatic (20–30%) and intrahepatic (10–20%) CCA [1,2]. Perihilar CCAs develop between the secondary branches of the intrahepatic duct and the cystic duct [3], while extrahepatic CCAs arise between the ampulla of Vater and the cystic duct [4]. Intrahepatic CCAs originate in the smaller bile ducts distal to the secondary intrahepatic branches [5].
Globally, the incidence of CCA is less than 6 per 100,000 individuals [6], but significant regional variations exist, with higher rates in Asian countries like South Korea, Thailand, and China (>6 per 100,000) compared to Western nations (0.35–2 per 100,000) [6]. Alarmingly, CCA incidence has increased worldwide in recent decades [7,8], particularly intrahepatic CCA, which has seen a 350% increase, compared to a 20% rise in extrahepatic CCA [2]. Established risk factors include primary sclerosing cholangitis, parasitic infections, biliary-duct cysts, and hepatolithiasis. Less definitive risk factors encompass inflammatory bowel disease, chronic liver conditions (cirrhosis, hepatitis B and C), obesity, diabetes, alcohol consumption, smoking, and genetic predispositions [6,9].
Despite advances in Cca Diagnosis and treatment, survival rates remain poor. US-based research indicated median overall survival rates of 8 months for extrahepatic and 4 months for intrahepatic CCA between 1973 and 2008 [2]. Consistent with these findings, a Thai study from 2009–2013 reported a high 1-year mortality rate of 81.7% [10]. CCA’s high fatality stems from its biological aggressiveness, presentation at advanced stages, and high recurrence rates post-treatment.
2. Clinical Presentation of CCA
2.1. Common Signs and Symptoms
Obstructive jaundice, characterized by yellowing of the skin and eyes, dark urine, pale stools, and pruritus (itching), is a hallmark symptom of extrahepatic CCA due to bile duct blockage. In contrast, intrahepatic CCA, particularly in its early stages, may not cause jaundice. Patients with intrahepatic CCA may present with non-specific symptoms like dull, persistent right upper quadrant abdominal pain, malaise, night sweats, unexplained weight loss, or cachexia (muscle wasting) [11]. Importantly, CCA can be incidentally discovered through routine blood tests showing abnormal liver function, even in asymptomatic individuals [12].
2.2. Physical Examination Findings
Physical examination in extrahepatic CCA may reveal jaundice, hepatomegaly (enlarged liver), and a palpable mass in the right upper abdomen [13]. Intrahepatic CCA patients often exhibit fewer physical signs, possibly only right upper quadrant tenderness. Courvoisier’s sign, a palpable gallbladder in a jaundiced patient, is suggestive of pancreaticobiliary malignancy but is not specific to CCA, as it can also occur in chronic pancreatitis, biliary obstruction, and choledochal cysts [14]. Rarely, CCA may manifest with cutaneous paraneoplastic syndromes such as Sweet syndrome [15], erythema multiforme [16], or porphyria cutanea tarda [17].
3. Laboratory Biomarkers in CCA Diagnosis
Liver function tests are crucial in the initial assessment of suspected CCA. Total and direct bilirubin, gamma-glutamyltransferase (GGT), alkaline phosphatase (ALP), and serum transaminases (aspartate aminotransferase [AST] and alanine aminotransferase [ALT]) should be evaluated to detect cholestasis. Extrahepatic CCA typically causes significant elevation in total bilirubin (often >10 mg/dL), direct bilirubin, ALP, and GGT, usually 2- to 10-fold above normal. Initially, AST and ALT may be normal, but persistent cholestasis can lead to hepatocellular damage, resulting in elevated transaminases and prolonged prothrombin time. Intrahepatic CCA often presents with elevated ALP, while serum bilirubin levels may be mildly elevated or within the normal range [13].
Key serum tumor markers for CCA diagnosis include carbohydrate antigen 19-9 (CA 19-9) and carcinoembryonic antigen (CEA). However, their diagnostic utility is limited by low sensitivity in early-stage CCA and potential elevation in benign biliary conditions. Alpha-fetoprotein (AFP) can aid in differentiating intrahepatic CCA from HCC.
3.1. CA 19-9 Tumor Marker
CA 19-9, a sialyl-Lewis antigen epitope, is produced by various epithelial cells, including those of the pancreaticobiliary duct [18]. As a diagnostic biomarker for CCA, CA 19-9 demonstrates a sensitivity ranging from 50–90% and a specificity of 54–98% [19,20,21]. Elevated CA 19-9 levels at diagnosis are associated with poorer prognosis [22,23], and markedly elevated levels (>1,000 units/mL) often indicate unresectable disease [20,24]. Furthermore, CA 19-9 can be useful in detecting CCA in patients with primary sclerosing cholangitis [25].
However, limitations of CA 19-9 include its lack of specificity, as it is frequently elevated in benign conditions like cholangitis or bile duct strictures. The optimal cutoff value for CA 19-9 to distinguish between malignant and benign biliary diseases varies depending on the presence of cholangitis or cholestasis [26,27]. Additionally, CA 19-9 may not be elevated in Lewis-antigen-deficient individuals (5–10% of the population) even with CCA [27].
3.2. CEA Tumor Marker
CEA, a cell membrane-associated glycoprotein, exhibits differential expression between normal and malignant tissues. Elevated serum CEA levels can be found in CCA patients. A CEA level above 5.2 ng/mL in CCA diagnosis showed a sensitivity of 68% and a specificity of 82% [28]. However, CEA alone is not sufficiently sensitive or specific for CCA detection, as it can be elevated in various benign conditions (peptic ulcer disease, gastritis, diverticulitis, liver disease) and other gastrointestinal cancers. CEA is more useful for monitoring treatment response and detecting recurrence when initial CA 19-9 levels are not elevated.
3.3. AFP Tumor Marker
Serum AFP levels should be measured in patients with liver lesions. AFP is primarily used in HCC and germ cell tumor diagnosis [29]. Studies have shown that elevated AFP levels (>20 ng/mL) are present in a subset of intrahepatic CCA patients (around 19%), with higher levels (>200 ng/mL and >1000 ng/mL) being less frequent [30]. AFP has high specificity for HCC but limited sensitivity and specificity for CCA.
3.4. Serum IgG4 Levels
IgG4-associated sclerosing cholangitis can mimic CCA. While the role of IgG4 testing in suspected CCA is not fully defined, measuring serum IgG4 levels may be considered if IgG4-related sclerosing cholangitis is suspected. It’s important to note that serum IgG4 can also be elevated in CCA patients [31].
4. Radiologic Imaging for CCA Diagnosis
4.1. Transabdominal Ultrasound (TAUS)
TAUS is typically the initial imaging modality for patients presenting with jaundice to confirm bile duct dilatation, identify obstruction causes, and exclude gallstones [32]. If TAUS does not reveal a benign cause of biliary obstruction, further imaging with cross-sectional techniques (CT or MRI), EUS, or ERCP is necessary. TAUS is highly sensitive in detecting biliary dilatation and assessing obstruction severity. Studies have reported sensitivities of 89% for detecting ductal obstruction and 94% for localizing the obstruction site [33]. TAUS is preferred for patients with right upper abdominal pain without jaundice due to its superior sensitivity for detecting gallstones and biliary dilatation compared to CT. However, when CCA suspicion is high, cross-sectional imaging is favored over TAUS.
TAUS findings in CCA can vary: hilar CCA (Klatskin tumor) may show bilateral intrahepatic duct nonunion and segmental dilatation; papillary tumors appear as polypoid masses within the biliary tract; nodular CCAs are seen as smooth, isolated masses with mural thickening; and intrahepatic CCA may present as a large mass with irregular margins and variable echogenicity depending on fibrosis, mucin, and calcification [34]. In the absence of stones, biliary dilatation (duct diameter >6 mm in individuals with intact gallbladder) suggests obstruction. Proximal extrahepatic CCAs might only dilate intrahepatic ducts, while distal CCAs can dilate both intra- and extrahepatic ducts [35]. Tumors often manifest at points of abrupt ductal diameter change. A limitation of TAUS is potential masking of the distal common bile duct by bowel gas. Biliary dilatation is often used as a surrogate marker for distal obstruction. In patients with PSC or cirrhosis, biliary dilatation may be less evident in CCA. However, progressive biliary dilatation in PSC patients with a dominant stricture should raise suspicion for CCA [36]. Duplex ultrasound can assess vascular involvement (encasement, compression, or thrombosis).
4.2. Multidetector Computed Tomography (MDCT)
MDCT is a widely available alternative to TAUS in suspected CCA cases. It is valuable for detecting intrahepatic malignancies, assessing biliary stenosis extent, and identifying liver atrophy. MDCT can also help differentiate malignant from benign intrahepatic bile duct strictures, particularly in the portal venous phase, and assess nodal involvement [37,38].
4.2.1. Intrahepatic CCA on MDCT
Intrahepatic CCA exhibits three main growth patterns: mass-forming (most common, ~60%), periductal-infiltrating, and mixed (each ~20%) [31]. In patients without cirrhosis, intrahepatic lesions are more likely to be metastases. In cirrhotic patients, differentiation from HCC is crucial. Intrahepatic CCA typically appears as a hypodense hepatic lesion, well-defined or infiltrative, with bile duct dilatation. Capsular retraction may be seen in ~20% of cases due to tumor fibrosis. Intrahepatic CCA often shows peripheral rim enhancement in arterial and venous phases [37,39]. Small mass-forming intrahepatic CCAs may mimic HCC with arterial hyperenhancement and venous washout [40].
Combined hepatocellular-cholangiocarcinoma (HCC-CCA) can have MDCT features distinct from both HCC and CCA, lacking typical differentiating patterns or biliary dilatation [41,42]. Biopsy is often required for pathological confirmation when typical HCC or CCA features are absent.
4.2.2. Extrahepatic CCA on MDCT
In extrahepatic and perihilar CCA, ductal dilatation patterns indicate lesion location. Perihilar CCA is suggested by dilatation of both intrahepatic ducts with separation of left and right hepatic ducts. Intrahepatic CCA, causing jaundice later, tends to be larger with liver parenchyma invasion. Intrahepatic ductal dilatation and lobar atrophy can suggest the origin site. Dilatation of intra- and extrahepatic ducts, plus gallbladder, suggests a periampullary tumor. Isolated gallbladder enlargement without duct dilatation suggests cystic duct malignancy or stones.
4.3. Magnetic Resonance Imaging (MRI) and MRCP
On MRI, CCA typically appears hypointense on T1-weighted images and heterogeneously hyperintense on T2-weighted images [43]. Central T2 hypointensity may indicate intratumoral fibrosis. Contrast enhancement shows gradual, concentric filling after peripheral enhancement, with delayed enhancement suggestive of peripheral CCA.
Mixed HCC-CCA has distinct MRI features [44,45,46]. Gadoxetic acid-enhanced MRI may show a strong enhancing rim and irregular margin in mixed HCC-CCA, while mass-forming intrahepatic CCA may show a lobulated shape, target appearance, and weak enhancing rim [45]. Target appearance can differentiate mixed HCC-CCA from atypical hypovascular HCC [46]. MRI with MRCP is non-invasive for biliary tract evaluation and preoperative CCA assessment. MRCP avoids bile duct contrast injection unlike ERCP. MRCP offers advantages over MDCT, including intrahepatic disorder visualization and 3D bile duct and vascular structure reconstruction.
MRCP provides disease extent and resectability information comparable to MDCT, ERCP, and angiography [47,48,49,50]. MRCP and ERCP showed 100% biliary obstruction detection in a perihilar CCA study, but MRCP excelled in anatomical extent and jaundice cause determination [51]. MRCP and MDCT can replace invasive cholangiography in proximal obstructive jaundice [52]. However, MRCP cannot perform interventions like biopsy or stenting. MRCP should be considered before biliary drainage as post-drainage collapsed ducts are harder to evaluate.
4.4. Positron Emission Tomography (PET) Scan
FDG-PET scanning is used for CCA staging, not primary tumor diagnosis, where MDCT or MRI with MRCP are superior. PET scans are useful for detecting occult metastases in potentially resectable CCA before surgery.
CCAs are usually FDG-avid due to bile duct epithelium glucose uptake [53]. PET/CT can detect nodular CCAs as small as 1 cm, but is less effective for infiltrating tumors with lower FDG uptake [54,55]. SUVmax and tumor-to-normal liver ratio may differentiate malignant from benign lesions [56,57], but definitive cutoff values are not established.
4.5. Chest CT
NCCN guidelines recommend chest CT for surgical CCA candidates during initial workup to assess for distant metastases [58].
5. Endoscopic Approaches to CCA Diagnosis
5.1. Cholangiography (PTC and ERCP)
Cholangiography, involving contrast injection into the biliary tract via percutaneous (PTC) or endoscopic (ERCP) routes, detects biliary abnormalities. While less invasive techniques like MRCP and MDCT have largely replaced diagnostic cholangiography [52], it remains valuable for histological confirmation and preoperative drainage. Some clinicians still prefer cholangiography over MRCP for assessing biliary obstruction extent and location. Extrahepatic CCA often spreads longitudinally along the bile duct, potentially leaving residual tumor at surgical margins. Percutaneous transhepatic cholangioscopy with mapping biopsies can improve preoperative longitudinal spread assessment, achieving 80–92% accuracy when combined with cholangiography [59,60,61].
PTC or ERCP choice depends on lesion location and patient factors. ERCP is preferred in PSC patients with difficult percutaneous access due to strictures. Novel devices like EndoSheather can facilitate mapping biopsies in hilar CCA and targeted stricture biopsies during ERCP, reducing pancreatitis risk and cancer cell contamination [62,63].
Brush cytology or biopsy via ERCP or PTC is crucial for tissue diagnosis. Meta-analysis shows diagnostic sensitivities of 56% for brushing, 67% for biopsy, and 70.7% for combined brushing and biopsy in CCA [64]. Combining elevated CA 19-9 with positive brush cytology yields 88% sensitivity and 97% specificity [28]. ERCP biopsy of extrahepatic CCA is illustrated in Figure 1.
5.2. Endoscopic Ultrasound (EUS)
EUS assesses regional lymph node status and local extent of extrahepatic CCA. While cholangiography remains primary for CCA diagnosis, EUS with FNA is clinically important, providing tissue samples from tumors and lymph nodes [65]. EUS-FNA is more sensitive than ERCP brushings for extrahepatic CCA diagnosis [66], minimizing biliary tree contamination and ensuring adequate tissue acquisition. EUS-FNA sensitivity varies with tumor location, but many studies report sensitivities over 70% for extrahepatic CCA, ranging from 43% to 89% [67,68,69,70,71]. EUS is less sensitive for staging intrahepatic CCAs [71,72]. EUS-guided FNA and biopsy in suspected intrahepatic CCA are shown in Figure 2.
5.3. Intraductal Ultrasound (IDUS)
IDUS uses a high-frequency ultrasound miniprobe within the biliary tract (12–30 MHz) [73]. IDUS distinguishes malignant from benign strictures based on sonographic features like eccentric wall thickening, irregular surface, hypoechoic mass, disrupted three-layer structure, heterogeneous echo pattern, and papillary surface [74]. IDUS improves local CCA staging, aiding in early tumor detection, longitudinal extent evaluation, and invasion assessment [75]. IDUS is more effective than EUS for proximal biliary system and surrounding structure evaluation, but has limited penetration depth for distant tissues or lymph nodes and cannot perform FNA.
5.4. Cholangioscopy (SOC)
Cholangioscopy visualizes the biliary tract using a thin cholangioscope inserted directly into the bile duct [76], via peroral or percutaneous routes. Oral route is generally preferred due to risks associated with the percutaneous approach. Cholangioscopy, with or without biopsy, improves diagnostic yield over ERCP alone. It is used for ambiguous ERCP findings, preoperative CCA extent evaluation, and detecting radiolucent stones. Cholangioscopy allows direct biliary epithelium visualization for targeted biopsies and lithotripsy [77,78]. Single-operator cholangioscopy (SOC) with biopsy shows up to 85% accuracy in diagnosing indeterminate strictures, outperforming cytology and ERCP-guided biopsy (34% and 54%, respectively) [77,79,80]. It also allows endobiliary instrument passage for advanced procedures. Cholangioscopic findings of hilar CCA are shown in Figure 3.
Table 1 summarizes the advantages and limitations of endoscopic modalities in CCA diagnosis.
Table 1. Advantages and limitations of endoscopic modalities for diagnosing cholangiocarcinoma.
Modality | Advantages | Limitations |
---|---|---|
ERCP | – Anatomic delineation of biliary strictures – Real-time visualization for biopsy/stenting | – Invasive – Incomplete proximal duct evaluation in high-grade obstruction |
EUS | – Detailed extrahepatic bile duct and surrounding structure examination – EUS-FNA/B for cytology/pathology | – Rare risk of tumor seeding during EUS-FNA/B |
SOC | – Direct biliary epithelium visualization – Targeted biopsy capability | – Technically challenging (requires significant sphincterotomy) |
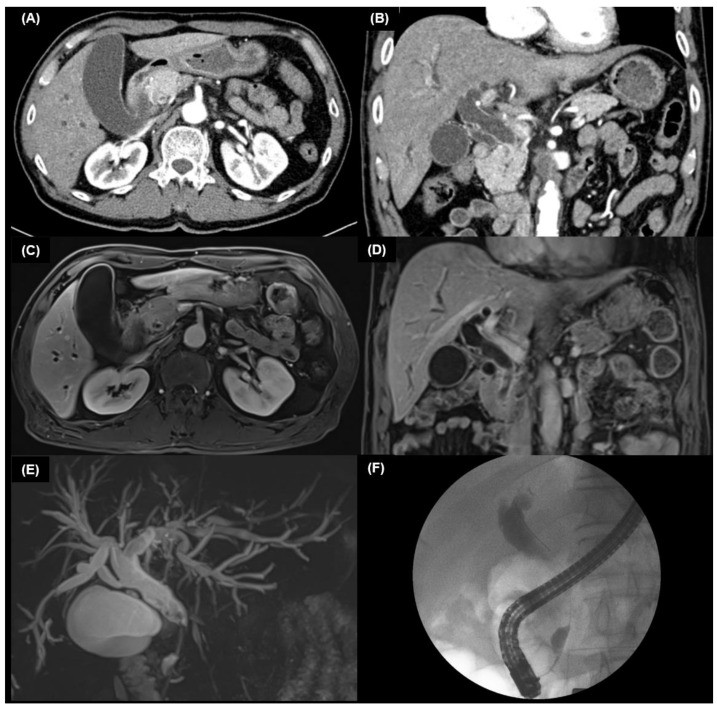
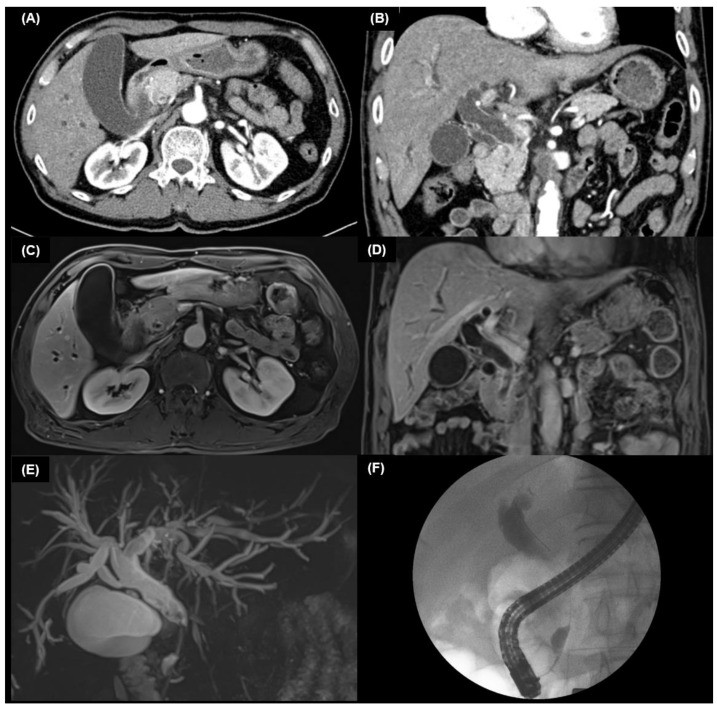
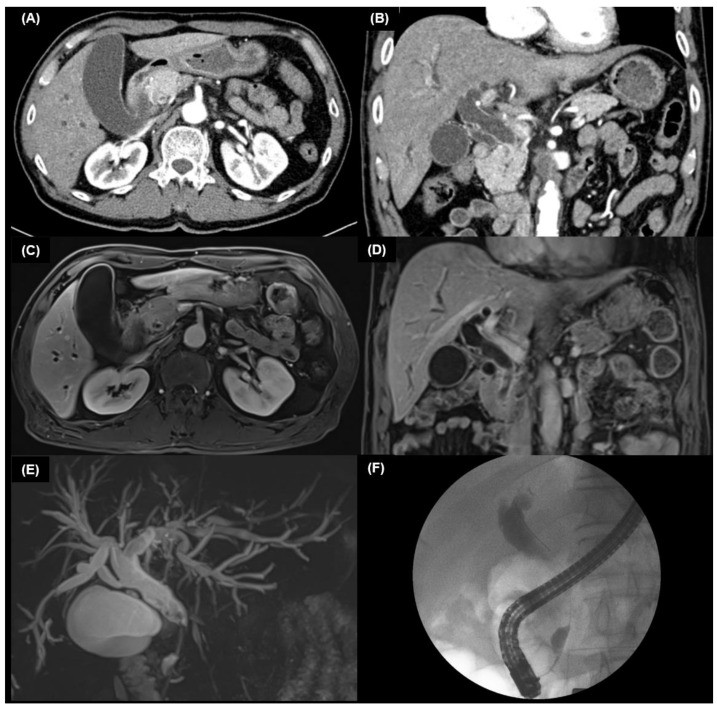
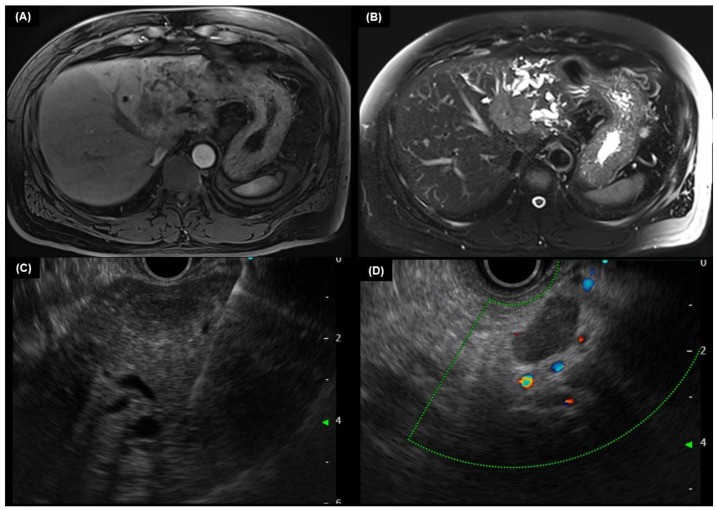
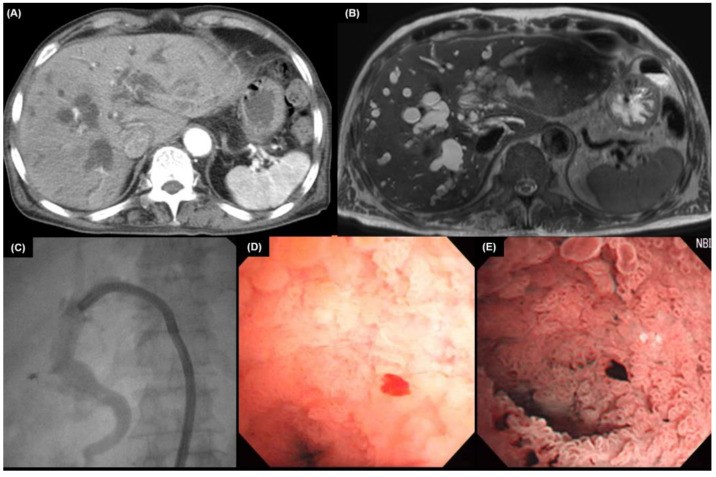
6. Pathological Examination of CCA
Histologically, intrahepatic CCAs are predominantly adenocarcinomas with varied microscopic patterns. Small-duct intrahepatic CCAs often mimic small biliary ductules, while large-duct intrahepatic and extrahepatic CCAs share features like tubular patterns, desmoplastic stroma, and perineural and lymphovascular invasion [6]. Immunohistochemistry aids in differentiating primary hepatic tumors [81]. CCAs typically show strong positivity for cytokeratin (CK)7 and CK19, consistent with biliary origin. However, metastatic lung and breast cancers can also be CK7 positive, making CCA a diagnosis of exclusion in some cases. CK20 positivity can be seen in up to 20% of intrahepatic CCA cases. An antibody panel including hepatocyte paraffin 1, arginase-1, monoclonal carcinoembryonic antigen, CK7, CK20, TTF-1, and CDX2 can optimize differentiation between HCC, metastatic adenocarcinoma, and CCA [82]. Ruling out HCC, which is variably positive for hepatocyte paraffin 1, glypican-3, and arginase-1, is clinically crucial.
7. Genomic Heterogeneity in CCA
CCA tumorigenesis is driven by diverse molecular alterations affecting pathways like DNA repair (TP53) [83,84], WNT–CTNNB1 [85], tyrosine kinase signaling (KRAS, BRAF, SMAD4, FGFR2) [86,87,88], protein tyrosine phosphatase (PTPN3) [89], epigenetic modifications (IDH1, IDH2) [83,84,90,91], and chromatin-remodeling factors (MLL3, SWI/SNF complex components ARID1A, PBRM1, BAP1) [83,84,90,91]. Deregulated Notch signaling, vital in cholangiocyte differentiation and biliary development, also plays a role.
8. Conclusion: Key Takeaways for CCA Diagnosis
Cholangiocarcinoma encompasses malignancies of the perihilar, extrahepatic, and intrahepatic bile ducts. Extrahepatic and perihilar CCA often manifest with asymptomatic jaundice, weight loss, and right upper quadrant discomfort, while intrahepatic CCA may lack jaundice.
Initial diagnostic imaging frequently involves cross-sectional scans like MDCT or MRI with MRCP. Extrahepatic CCA typically shows abrupt ductal diameter changes with dilatation of both intra- and extrahepatic ducts. Perihilar CCA presents with intrahepatic duct dilatation and normal-sized extrahepatic ducts. Intrahepatic CCA is often identified as a mass lesion in non-cirrhotic livers.
Tumor biomarkers (CA 19-9, CEA) should be assessed in all suspected CCA cases, with AFP additionally checked for intrahepatic tumors. These biomarkers aid in diagnosis, treatment monitoring, and recurrence detection.
For extrahepatic CCA, EUS or ERCP is recommended for further evaluation, enabling tissue acquisition (EUS-FNA or ERCP) and therapeutic interventions like stent placement (ERCP). Perihilar lesions are initially assessed with MDCT or MRCP, followed by ERCP for histological confirmation. Intrahepatic lesions require differentiation from HCC using imaging and tumor markers. PET scans are recommended for surgical candidates without distant metastases.
Author Contributions: Conceptualization, J.H.K.; methodology, J.H.K.; writing—original draft preparation, D.W.S.; writing—review and editing, S.-H.M.; visualization, D.W.S.; supervision, J.H.K. All authors have read and agreed to the published version of the manuscript.
Conflicts of Interest: The authors declare no conflict of interest.
Funding Statement: This research received no external funding.
Disclaimer/Publisher’s Note: The statements, opinions and data contained in all publications are solely those of the individual author(s) and contributor(s) and not of MDPI and/or the editor(s). MDPI and/or the editor(s) disclaim responsibility for any injury to people or property resulting from any ideas, methods, instructions or products referred to in the content.
References
[1] Khan, S.A.; Tavolari, S.; Brancaleone, V.; Valle, J.W. Cholangiocarcinoma: Epidemiology and risk factors. Gut Liver 2010, 4, 425–432.
[2] Patel, T. Cholangiocarcinoma: Epidemiology, risk factors, and clinical presentation. Liver Int. 2011, 31 (Suppl. 1), 7–15.
[3] Blechacz, B. Cholangiocarcinoma: Classification and staging. Hepatobiliary Surg. Nutr. 2018, 7, 396–401.
[4] Nakeeb, A.; Pitt, H.A.; Sohn, T.A.; Coleman, J.; Abrams, R.A.; Piantadosi, S.; Hruban, R.H.; Lillemoe, K.D.; Yeo, C.J.; Cameron, J.L. Cholangiocarcinoma. A spectrum of intrahepatic, perihilar, and distal tumors. Ann. Surg. 1996, 224, 463–473; discussion 473–475.
[5] Razumilava, N.; Gores, G.J. Cholangiocarcinoma. Lancet 2014, 383, 2168–2179.
[6] Banales, J.M.; Marin, J.J.G.; Lamarca, A.; Rodrigues, P.M.; Khan, S.A.; Roberts, L.R.; Cardinale, V.; Gurusamy, K.S.; Davidson, B.R.; Braconi, C.; et al. Cholangiocarcinoma 2020: The next horizon in mechanisms and management. Nat. Rev. Gastroenterol. Hepatol. 2020, 17, 481–500.
[7] Shaib, Y.H.; El-Serag, H.B. The epidemiology of cholangiocarcinoma. Semin. Liver Dis. 2004, 24, 115–125.
[8] Welzel, T.M.; McGlynn, K.A.; Hsing, A.W.; O’Brien, T.R.; Pfeiffer, R.M.; El-Serag, H.B.; Davila, J.A.; Anderson, K.E. Impact of classification of hilar cholangiocarcinoma (Klatskin tumors) on the incidence trends for intra- and extrahepatic cholangiocarcinoma in the United States. J. Natl. Cancer Inst. 2006, 98, 873–875.
[9] Tyson, G.L.; El-Serag, H.B. Risk factors for cholangiocarcinoma. Hepatology 2011, 54, 173–184.
[10] Khuntikeo, N.; Titapun, A.; Bhudhisawasdi, V.; Pairojkul, C.; Petrakorn, S.; Khuntikeo, P.; Promthet, S.; Parkin, D.M. High mortality of cholangiocarcinoma: A population-based study in Thailand. Liver Int. 2016, 36, 246–252.
[11] Bergquist, A.; von Seth, E. Epidemiology of cholangiocarcinoma. Best Pract. Res. Clin. Gastroenterol. 2015, 29, 221–232.
[12] Mavilia, M.G.; Wu, G.Y. Cholangiocarcinoma: Diagnosis and management. World J. Gastroenterol. 2021, 27, 4724–4744.
[13] O’Connor, S.; Cashman, S.; Linehan, J.; Sweeney, E.C.; O’Morain, C.A.; Hegarty, J.E. Cholangiocarcinoma: Diagnostic and therapeutic dilemmas. Ir. J. Med. Sci. 2007, 176, 19–23.
[14] Charoensuk, C.; Lee, W.M.; Phisalprapa, P.; Charatcharoenwitthaya, P. Courvoisier’s sign in biliary obstruction by stones and malignant causes: A prospective observational study. Hepatobiliary Pancreat. Dis. Int. 2017, 16, 531–535.
[15] Cohen, P.R. Sweet’s syndrome—A comprehensive review of an acute febrile neutrophilic dermatosis. Orphanet J. Rare Dis. 2007, 2, 34.
[16] Wolf, R.; Ruocco, V.; Vescovini, P.; Verona, E. Erythema multiforme. J. Am. Acad. Dermatol. 2004, 51, 788–796.
[17] Todd, D.J.; Tomcik, M.; Warshawsky, H.; Grossman, M.E. Porphyria cutanea tarda and cholangiocarcinoma. J. Am. Acad. Dermatol. 2004, 51, 822–824.
[18] Gold, P.; Freedman, S.O. Specific carcinoembryonic antigens of the human digestive system. J. Exp. Med. 1965, 122, 467–481.
[19] Vach, K.; Vehling-Kaiser, U.; Vogl, T.J.; Engelbach, J.A.; Golchert, J.; Stenger, A.; Weinmann, A.; Hoffmann, R.T. Diagnostic accuracy of carbohydrate antigen 19-9 and carcinoembryonic antigen in patients with suspected cholangiocarcinoma. BMC Cancer 2018, 18, 840.
[20] Patel, K.; Kim, Y.; Bridgewater, J.; Marples, M.; Hebbar, M.; Mukherjee, S.; Valle, J.; Armstrong, A.; Maishman, T.; Fernando, M.; et al. Serum CA19-9 in potentially resectable and unresectable cholangiocarcinoma: Prognostic and diagnostic value. J. Gastrointest. Oncol. 2019, 10, 255–265.
[21] Poruk, K.E.; Weber, S.M.; Rocha, F.G.; McGlynn, K.A.; Hughes, K.A.; Pawlik, T.M. Serum CA 19-9: Utility in predicting prognosis after resection of pancreatic adenocarcinoma and cholangiocarcinoma. J. Gastrointest. Surg. 2013, 17, 151–158; discussion 158–159.
[22] Anderson, C.D.; Parray, F.; Kim, Y.; Rocha, F.G.; Margonis, G.A.; Weiss, M.; Tran, T.B.; Bauer, T.W.; Pawlik, T.M. The role of serum CA 19-9 in predicting survival in patients with cholangiocarcinoma: A systematic review and meta-analysis. HPB 2016, 18, 273–281.
[23] Juntermanns, B.; Vangerow, B.; Bogoevski, D.; Hardel, S.; Marx, A.; Izbicki, J.R.; Klupp, J. Serum carbohydrate antigen 19-9 in patients with cholangiocarcinoma: Prognostic value and correlation with clinico-pathological parameters. Eur. J. Surg. Oncol. 2016, 42, 125–131.
[24] Kamiyama, H.; Yokoo, H.; Kamachi, H.; Wakayama, K.; Nagashima, K.; Taketomi, A. Clinical significance of preoperative carbohydrate antigen 19-9 levels in patients with perihilar cholangiocarcinoma. J. Hepatobiliary Pancreat. Sci. 2016, 23, 500–508.
[25] Chapman, R.; Fevery, J.; Kleiner, D.; MacCartney, J.; Milanowski, J.; Petzold, P.; Reynaert, H.; Rosenqvist, G.L.; Schrumpf, E.; Trauner, M.; et al. Cholangiocarcinoma and primary sclerosing cholangitis. Hepatology 2010, 51, 1–22.
[26] Boonstra, K.; Weersma, R.K.; van Erpecum, K.J.; van Milligen de Wit, A.W.; Spanier, B.W.; Poen, A.C.; Witteman, B.J.; Huibregtse, K.; Offerhaus, G.J.; van Gulik, T.M.; et al. Serum CA 19-9 in the diagnosis of cholangiocarcinoma in primary sclerosing cholangitis patients: A systematic review. Liver Int. 2012, 32, 943–950.
[27] Patel, A.H.; Davila, J.A.; El-Serag, H.B. Serum levels of CA 19-9 and CEA in the diagnosis of cholangiocarcinoma: A systematic review and meta-analysis. Clin. Gastroenterol. Hepatol. 2008, 6, 413–420.
[28] Kaufman, M.S.; El-Khoueiry, A.B.; Chen, K.; McMillan, A.; Sze, D.Y.; Venook, A.P.; Kelley, R.K. Utility of serum carbohydrate antigen 19-9 and carcinoembryonic antigen levels in combination with endoscopic retrograde cholangiopancreatography-guided brush cytology for the diagnosis of cholangiocarcinoma. Pancreas 2014, 43, 430–437.
[29] Marrero, J.A.; Feng, Z.; Wang, Y.; Nguyen, M.H.; Chen, Y.; Reddy, K.R.; Ahn, J.C.; Rajender, J.; Llovet, J.M. Alpha-fetoprotein, des-gamma carboxyprothrombin, and lectin-bound alpha-fetoprotein in early hepatocellular carcinoma versus chronic liver disease. Gastroenterology 2009, 137, 110–118.
[30] Oishi, K.; Itai, Y.; Kuba, S.; Kokubo, T.; Tanaka, Y.; Minami, Y.; Yamauchi, R.; Nakanuma, Y.; Honda, H.; Miyakawa, S. Clinicopathologic features of intrahepatic cholangiocarcinoma with elevated serum alpha-fetoprotein levels. Am. J. Gastroenterol. 2000, 95, 1702–1707.
[31] Zen, Y.; Harada, K.; Sasaki, M.; Sato, Y.; Niwa, H.; Fujii, T.; Abe, Y.; Takemura, A.; Miyamoto, Y.; Kasashima, S.; et al. IgG4-related sclerosing cholangitis with and without hepatic inflammatory pseudotumor and sclerosing pancreatitis-associated hyper-IgG4 disease. Am. J. Surg. Pathol. 2004, 28, 1191–1203.
[32] Barreto, S.G.; Gupta, R.; Gulati, A.; Patel, K.; Phillipraj, S.A.; Rathi, P.; Goel, A.; Kumar, R.; Pal, S.; Chaudhary, A. Role of ultrasound in biliary obstruction. Indian J. Gastroenterol. 2013, 32, 282–290.
[33] Dewbury, K.C.; Birch, S.J. Ultrasound in obstructive jaundice. Br. J. Radiol. 1980, 53, 635–641.
[34] Choi, B.I.; Lee, J.K.; Kim, S.H.; Kim, K.W.; Kim, A.Y.; Lee, J.Y.; Han, J.K.; Kim, D.Y.; Choi, D.W. Hilar cholangiocarcinoma: US and CT findings. Abdom. Imaging 2000, 25, 501–507.
[35] Albrecht, T.; Romaniuk, P.; Seifert, G.; Mahlke, R.; Beyer, J.; Hirner, A.; Rogiers, X.; Broelsch, C.E.; Felix, R. Biliary obstruction due to malignant strictures: Value of spiral-CT cholangiography. Eur. Radiol. 2000, 10, 939–944.
[36] Lee, K.S.; Sekijima, J.H.; Callery, M.P.; Christein, J.D.;ложено